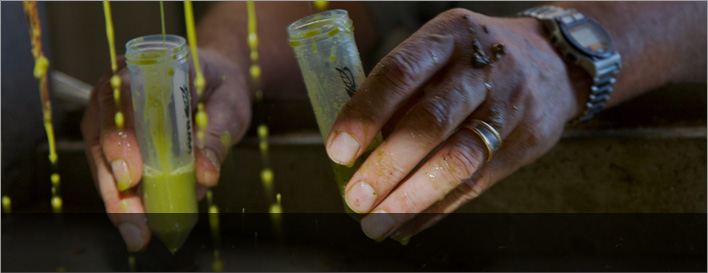
2008-2013: Oklahoma NSF EPSCoR Cellulosic BioEnergy Research
Biofuels: Innovation for our Energy Future
** Archived Project **
(This initiative is no longer active in our portfolio.)
Oklahoma scientists and engineers are developing unique methods to transform non-crop native grasses, such as switchgrass, into liquid fuel. Project leadership is provided by researchers from Oklahoma State University, University of Oklahoma and Samuel Roberts Noble Foundation.
- View the research team
- View the informational flier
- View a collection of Oklahoma NSF EPSCoR research videos
Ethanol can be generated by microbial fermentation from a variety of substrates including starch and lignocellulose. However, the availability of sugars for ethanol production from such crops is limited because of the value of the feedstocks for the food industry. Lignocellulose, in comparison, is inexpensive and plentiful. Thus, there is great interest in using lignocellulose as a renewable energy source for ethanol production through microbial fermentation or for conversion to liquid fuels via pyrolysis and gasification.
The goal of the EPSCoR research effort is to develop enhanced methods to extract biofuels from switchgrass. Briefly, the specific aims are:
- Enhance biomass yield using genetic engineering and other plant breeding techniques
- Enhance the conversion of biomass into biofuels by microbial fermentation
- Enhance the conversion of biomass into biofuels by thermocatalysis
Research Objectives
Objective 1To discover molecular mechanisms and tools for biomass development by genomics, functional genomics and genetic transformation approach.Click this tab to view information about this objective.
Perennial and high yielding grasses, such as switchgrass (Panicum virgatum L.), Miscanthus spp, eastern gamagrass (Tripsacum dactyloides), and Bermuda grass (Cynodon dactylon) can be developed into dedicated biofuel crops. Switchgrass, for example, is a tallgrowing plant native to Oklahoma and tops the perennial list. The grass has been chosen as the model herbaceous species as a feedstock energy crop because of its potential high biomass yield on marginal land, wide adaptation, and positive environmental attributes.
Understanding molecular mechanisms of biomass production, improvement of its quality in the grass, and the development of molecular tools to improve grass breeding efforts are likely to increase biomass yield potential. It may also enhance tolerance to abiotic and biotic stresses, and improve feedstock quality directionally for enhancing the conversion process of biomass to ethanol.
Achievement of these goals would improve the economy of a viable biofuel industry based on cellulosic biomass production. Accordingly, we plan to elucidate the mechanisms of biomass accumulation in dedicated biofuel grasses, and to develop new genetic improvement tools for biomass production by genomics, functional genomics, and genetic transformation approaches. To achieve the objective, the following four specific aims will be pursued.
Aim 1.1
To analyze gene expression associated with biomass accumulation
Leader: Mali Mahalingam (OSU)
Aim 1.2
To construct a DNA marker genetic map and identify major QTLs for key biomass components
Leader: Yanqi Wu (OSU)
Aim 1.3.a
To identify and characterize microRNAs important for biomass accumulation and stress tolerance
Leader: Ramanjulu Sunkar (OSU)
Aim 1.3.b
To understand the molecular basis of total biomass production by dissecting key developmental programs and subsequently manipulate biomass for biofuel feedstock improvement
Leader: Million Tadege (OSU)
Aim 1.4.a
To identify genes involved in non-host resistance against Puccinia emaculata, the leaf and stem rust disease causing pathogen of switchgrass
Leaders: Kiran Mysore and Stephen Marek (OSU)
Aim 1.4.b
To develop a highly efficient switchgrass genetic transformation system and to produce transgenic switchgrass plants with improved biomass traits
Leader: Zeng-yu Wang (OSU)
Resources: Oklahoma is an ideal state to implement the RII project. The established grass breeding programs at OSU and SRNF have been used for biofuel crop breeding and genomics research.
Knowledge and molecular tools developed from the proposed RII project can be directly incorporated into the grass breeding efforts. With RII funding we can develop the infrastructure to address these questions.
The OSU Microarray Core Facility, established with RII support, and the Forage Improvement Division and Plant Biology Division of the SRNF have state-of-the-art facilities for plant molecular biology, genetic transformation, biochemistry and grass genetic improvement research. See “Facilities, Equipment, and Other Resources” for details.
Expected Impact: Selection of perennial grasses, like switchgrass, as dedicated biofuel crops is a relatively recent event and more knowledge of molecular mechanisms of cellulosic biomass accumulation and regulation is needed.
This project will provide a platform to conduct research aimed at discovery of the molecular mechanisms and development of knowledge to improve cellulosic biomass production and to further understand the biological mechanisms that control biosynthesis of cell wall components in grasses. Knowledge discovered will be useful in the development of cellulosic biomass production for biofuels.
Objective 2To understand the molecular basis and mechanisms underlying efficient microbial conversion of biomass to liquid fuels through direct and indirect fermentation.Click this tab to view information about this objective.
Despite extensive advances in the effective utilization of lignocellulose as a source of biofuels, various problems associated with practical use of these complex materials remain.
In the Consolidated BioProcessing (CBP) configuration, all required enzymes including fermentation to ethanol are produced by a single microbial community in a single reactor. The greatest advantage of CBP is the reduction in process steps and complexity of the reactor systems.
CBP presents an opportunity for efficient lignocellulosic ethanol production. So far, however, no microorganism or compatible combinations of microorganisms are available that both produce cellulose and other enzymes at the required high levels and also produce ethanol at the required high yields.
In the second objective, we will use various omics approaches to understand the molecular basis and mechanisms underlying efficient microbial conversion of biomass to liquid fuels through direct as well as indirect fermentation. To achieve the objective, the following four specific aims will be pursued.
Aim 2.1
Identification of the core genomes of Clostridia and Thermoanaerobacter, and identification of the core pathways of cellulose degradation and ethanol formation
Leaders: Zhili He, Chris Hemme, Jizhong Zhou (OU)
Aim 2.2
Compare gene expression profiles between the co-culture and individual monoculture under standard growth condition
Leaders: Zhili He, Chris Hemme, Jizhong Zhou (OU)
Aim 2.3.a
To investigate ecophysiology of acetogens converting syngas to ethanol
Leader: Ralph Tanner (OU)
Aim 2.3.b
Leader: Bradley Stevenson (OU)
Aim 2.4
To investigate the ecology, physiology and metabolic capabilities of anaerobic fungi providing an analysis on selected candidates in lignocellulosic biomass conversion
Leader: Mostafa Elshahed (OSU)
Resources: Through previous RII support, we have developed genome characterization and microbial ecology infrastructure. Our capabilities include facilities for research on model microbial organisms, environmental samples, and microbial community analyses on a genomic and/or meta-genomic scale.
Expected Impacts: Knowledge of the genomes and evolution of cellulose-degrading bacteria and ethanol-producing bacteria are important for manipulation of microorganisms for effective microbial conversion of plant biomass to liquid fuels. The study on comparative genomics of cellulolytic and noncellulolytic ethanol-producing bacteria will provide insights on their evolutionary processes, patterns and mechanisms.
Combining hydrolysis of cellulose with simultaneous fermentation of hexose and entose in a single process, i.e. Consolidated BioProcessing (CBP), is the most attractive strategy for converting cellulosic biomass to ethanol. However, no single microorganism or community is available for CBPbased strategy.
The information on the co-culture systems responding to different stresses at the genome-wide scale is also expected to provide insights on how to develop, improve and control the systems for efficient ethanol production. Such biotechnology will greatly benefit bioenergy studies and could potentially be transferred to bioenergy companies for biofuel production. Also, linking genomics to community/ecosystem dynamics, processes and functions is a major unaddressed biological question.
The research on establishing mechanistic linkages of molecular-level interactions/processes to population-level processes and functions is designed to address this question. Novel insights into whether and how the changes at the molecular levels affect population-level structure, functions and stability will be obtained.
This study will provide insights on how to scale and synthesize information across different organization levels for understanding the impacts of microbial diversity on community functioning and dynamics. These results will have a major impact on systems biology. In addition, the studies on understanding the pathways, enzymes and regulations in converting syngas to ethanol will facilitate developing such systems for industrial applications of this process for biofuel production.
Objective 3To improve existing and develop new catalytic/thermochemical conversion processes of cellulosic biomass.Click this tab to view information about this objective.
While biological processes for conversion of cellulose to ethanol have received considerable attention recently, thermocatalytic processes are an equally viable route for producing liquid fuels and chemicals from cellulosic biomass. In order to obtain liquid fuels or chemicals, the solid biomass usually undergoes a two step conversion; the first to obtain a liquid or gaseous intermediate, while the second step produces the desired molecules.
The primary focus of the biofuels work is on catalytic conversion of oxygenated compounds (Aims 3.1 – 3.3) with a secondary focus on heterogeneous catalysis in gasification and pyrolysis (Aim 3.4). Our interests and capabilities range from fundamental experimental studies on model catalysts with the goal of gaining a molecular-level understanding of the relevant reaction; to theoretical studies of catalyst-adsorbate and catalyst-support interactions; to characterization of fuel combustion properties. Significantly and, we believe, uniquely, our group proposes to develop QSAR (quantitative structure-activity relationship) models to predict specific properties (cetane, octane, sooting tendency, stability and fungibility) of biomass-derived fuel compounds and link these predictions with the distribution of products from a given catalyst which our catalytic studies give us. A key element is understanding how to convert compounds containing active oxygen moieties into stable and fungible fuel molecules or chemicals, while minimizing “counter-productive” chemistry (hydrogen consumption, loss of carbon, etc).
We believe there is now the opportunity to develop tailored heterogeneously catalyzed reactions to obtain fuels or chemicals of desired properties from a given biomass feedstock. This opportunity will be aggressively pursued through the four research aims below.
Aim 3.1
To develop catalysts and catalytic processes for conversion of oxygenated hydrocarbons to fungible fuels and chemicals
Leaders: Friederike Jentoft, Lance Lobban, Richard Mallinson, Daniel Resasco, Alberto Striolo (OU)
Aim 3.1.a
Oxygenates conversion
Leaders: Friederike Jentoft, Lance Lobban, Richard Mallinson, Daniel Resasco, Alberto Striolo (OU)
Aim 3.1.b
Leaders: Friederike Jentoft, Lance Lobban, Richard Mallinson, Daniel Resasco, Alberto Striolo (OU)
Aim 3.1.c
Studies of homogeneous catalytic conversion
Leaders: Friederike Jentoft, Lance Lobban, Richard Mallinson, Daniel Resasco, Alberto Striolo (OU)
Aim 3.2
To develop quantitative relationships between molecular structure and chemical properties of reactant and product molecules
Leaders: Friederike Jentoft, Lance Lobban, Richard Mallinson, Daniel Resasco, Alberto Striolo (OU)
Aim 3.3
Develop fundamental models of catalyst-support interactions, adsorbate-catalyst interactions and catalytic reactor behavior
Leaders: Friederike Jentoft, Lance Lobban, Richard Mallinson, Daniel Resasco, Alberto Striolo (OU)
Aim 3.3a (theoretical) and 3.3b (experimental)
Develop fundamental models of catalyst-support interactions, adsorbate-catalyst interactions and catalytic reactor behavior
Leaders: Friederike Jentoft, Lance Lobban, Richard Mallinson, Daniel Resasco, Alberto Striolo (OU)
Aim 3.4.a
To improve biomass gasification and pyrolysis processes through use of heterogeneous catalysts
Leaders: Friederike Jentoft, Lance Lobban, Richard Mallinson, Daniel Resasco, Alberto Striolo (OU)
Leaders: Daniel Bellmer, Ajay Kumar, Krushna Patil, Ray Huhnke (OSU)
Expected Impact: Existing processes for conversion of cellulosic biomass to fuels all have drawbacks and limitations. Hydrolysis-fermentation processes enjoy high selectivity but relatively slow kinetics requiring large residence times; gasification followed by Fischer-Tropsch conversion to diesel is economical only on a large scale, while the hydro treating of bio-oil requires large amounts of hydrogen and large scale hydro treating technology typically available only at petroleum refineries (and hydrogen availability is not guaranteed even there, given the high hydrogen demands of treating ever heavier crudes). The research proposed here develops another option for utilization of biomass. This option takes advantage of the reactivity of the oxygenated molecules to build larger molecules from small, and converts oxygenated molecules to more desirable fuel molecules or valuable chemicals using a minimum of hydrogen. Our studies will enable prediction of product distributions using various catalysts and the fuel properties of the product mixtures; this information will be fed back to enable optimization of conversion processes.